Warming of the Stratosphere Leads to Arctic Temperatures!
Temperatures in the two-digit minus range dominate the weather these days; a record-breaking seasonal value below -30°C was measured on Mount Zugspitze. People catch colds, automobiles do not start up, high-tension power lines break. What we are experiencing these days impressively demonstrates how the higher layers of Earth’s atmosphere can influence the weather on the ground – for scientists at EOC a fascinating field of research!
Normally, a vortex forms over the cold North Pole in winter. At its edge, especially strong wind currents prevail, extending from the upper troposphere (ca. 10 km altitude) up into the stratosphere (20 – 50 km altitude). They usually flow eastward. This wind obstructs the exchange of air masses between the warm equator and the cold pole, so it can be especially cold inside the vortex.
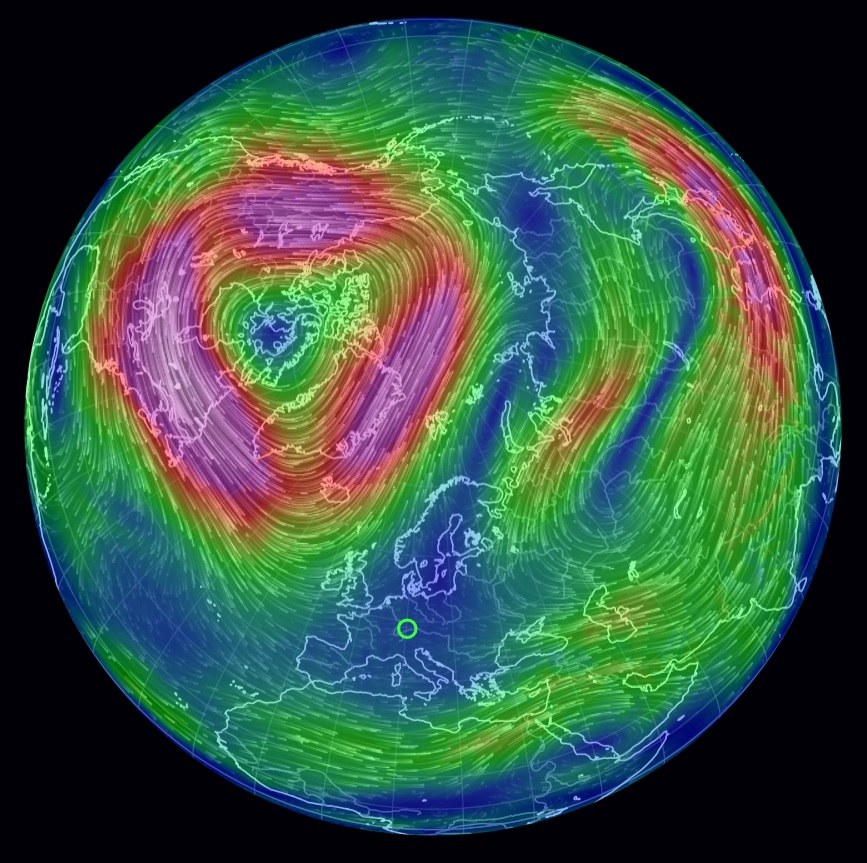
https://earth.nullschool.net/
During the past few weeks this eastward tending air flow underwent a complete change. Within a few days a break formed in the polar vortex permitting the diversion of ice cold air masses from the high north, heading to Europe as if on a conveyor belt. This situation continued for several days. The reason for this abrupt change in the global flow pattern is the warming of the stratosphere.
Again and again, the air currents spanning our planet encounter barriers: mountain chains, land-ocean transitions, and areas with intensified convection, such as the Gulf of Mexico. The zonal flow that is normally oriented along a circle of latitude is diverted southward or northward by these barriers. And this has serious consequences. It is one of the principles of physics that the angular momentum of an otherwise undisturbed system must be preserved. This is the why a moving bicycle or a spinning top rotating at a sufficient speed does not simply tip over.
The angular momentum of Earth gives rise to the Coriolis force, which changes with geographic latitude. This force causes an air packet that has been deflected northward (southward) to be diverted eastward (westward) with respect to the surface. In order to satisfy the principle of the preservation of angular momentum, the air masses have to oppose this change of planetary angular momentum. That occurs when the atmosphere generates a local angular momentum in the flow to re-establish the balance. As a consequence, the diverted air masses reverse their direction of flow and return to their original geographic latitude. Now another fundamental physical principle goes into effect: the sluggishness, or to use Isaac Newton’s designation, the inertia of a mass. As a result, the flow overshoots the position of its original geographic latitude and the opposite process goes into effect. The result is a flow of air oriented eastward that meanders back and forth across its original geographic circle of latitude. Physically, this flow pattern is an oscillation in space and time: a wave. Because of the planetary dimensions of these waves, the term planetary waves or Rossby waves is used for them. Rossby succeeded in producing the first mathematical description of essential structural characteristics of these waves in 1939.
Many such planetary waves are initiated in the atmosphere, and they have many different wavelengths and periods. The atmosphere has the amazing property of selecting only a few planetary waves from this broad spectrum and attenuating the rest. The remaining planetary waves can interact with each other, for example, gaining strength by overlapping and sometimes massively amplifying. Because of this resonance the wave field can become unstable within a few days and break. This is just like water waves gaining in amplitude as they reach the shore and then breaking as they form a whitecap. At that moment the energy stored in the wave field is released to its immediate surroundings. This can also be observed in daily life, a cork floating on water only rises and sinks when it encounters a water wave, but it remains at approximately the same location. However, if the wave breaks, the cork is cast away by the energy released from the wave field.
Something similar took place in the atmosphere over the past few weeks. The amplification of planetary waves led to a rapid, excessive increase in the wave field and thus to its eventual instability. When the wave field finally broke, the energy released split apart the cold polar vortex. This can be observed in the image of the atmospheric wind field (Fig. 1) and in satellite based measurements of the nitric acid (HNO3) concentration. Since HNO3 has a relatively long lifetime in the atmosphere it can be used as a marker to reveal air flows.

WDC-RSAT
Figure 2 shows how the breaking up of the vortex is reflected in the HNO3 concentration. It can be seen that the two parts over the pole were shoved away toward lower geographic latitudes. The cold air masses over the pole were replaced by warmer air masses. Because this process took place in the stratosphere one speaks of stratosphere warming. It changes the circulation so much that the effects extend down to the troposphere where they massively influence our weather.
But stratosphere warming also affects the mesopause region (80 – 90km). So warming of the stratosphere correlates with cooling in der mesosphere. This is shown by the daily temperature measurements at about 87 km elevation that are made by EOC with the GRIPS-12 equipment at the Haute Provence station in France (Fig. 3). It can be seen that there was a definite drop in temperature in February 2018, which, in addition, varied to an unusual extent. The modulation of the temperature can be traced back to the strong activity of planetary waves, which typically have a periodicity of several days.
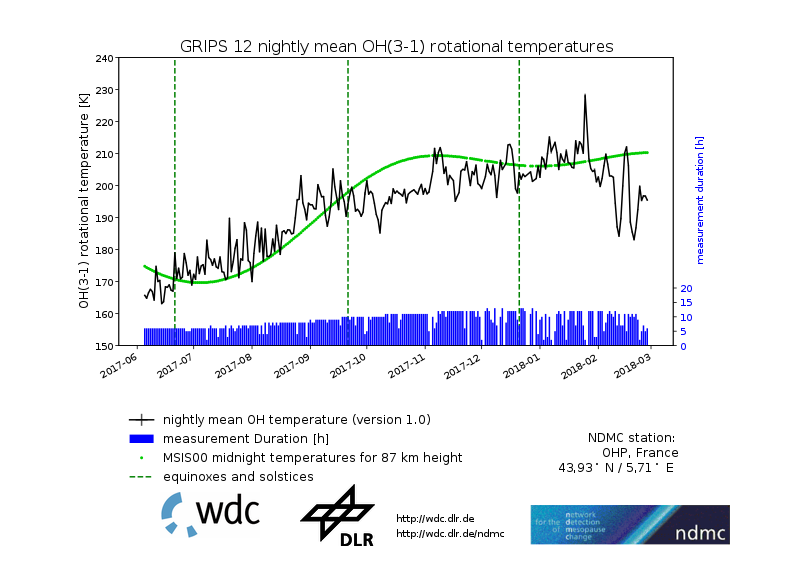
EOC scientists are investigating the conditions under which particular constellations of planetary waves can lead to extreme weather phenomena. A relevant new third-party funded project (WAVE) will begin already this year in cooperation with the Institute of Physics at Augsburg University. Besides its value for forecasting extreme weather situations, such knowledge is also of considerable scientific value. There are indications that a change in temperature distribution on our planet—for instance as a consequence of global warming—should influence the structure of planetary waves. Therefore, such extreme weather events could in the future change in frequency of occurrence and/or in intensity. Understanding how this happens and whether it is possible to detect such processes early also with the help of satellite-based measurements is a goal of EOC research efforts.