Quality matters – Advances in digital quantum simulation
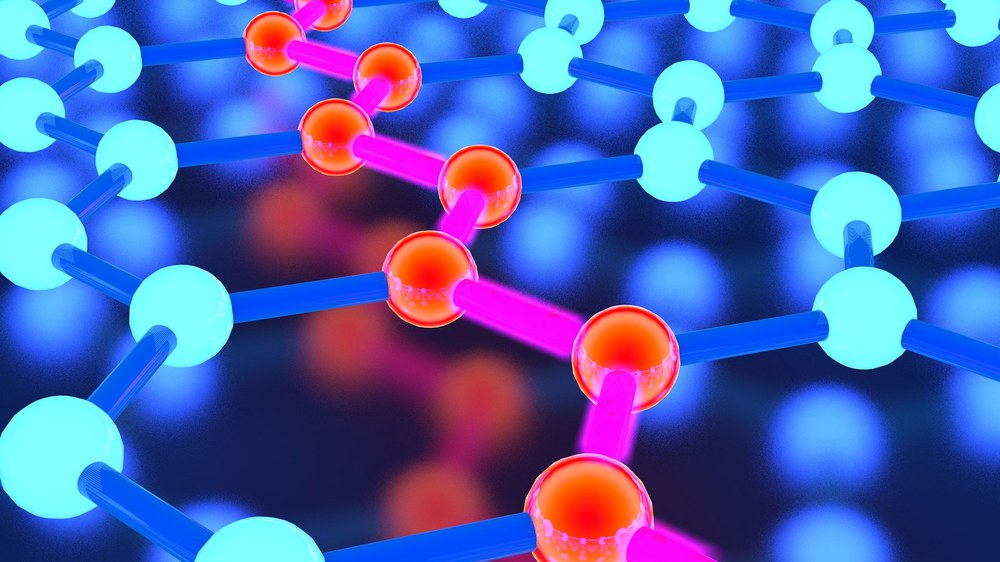
stock.adobe.com
- Digital quantum simulations can be used to solve problems in solid-state research, for example conductivity, magnetism or superconductivity.
- A quantum computer is required to carry out the simulations.
- Benedikt Fauseweh from the DLR Institute for Software Technology has analysed use cases.
- Focus: Digitalisation, quantum technologies, quantum computing, quantum advantage, fundamental research
All objects consist of atoms, which are made up of protons and neutrons in the atomic nucleus, surrounded by electrons. Once one enters this universe of the smallest things – the quantum world – it all gets very complicated very quickly. When researchers want to know exactly how atoms are connected and form solid objects, they come up against limits. No computer is powerful enough to calculate material properties precisely when many different atoms are involved. A researcher from the German Aerospace Center (Deutsches Zentrum für Luft- und Raumfahrt; DLR) has now investigated a way to do this via digital quantum simulation. This requires a quantum computer with initially only a few functioning computing and memory units, qubits, which could already answer questions about materials research – using quantum computing to ultimately even improve itself.
"In contrast to problems that can be solved with increased computing power alone, the complexity of quantum systems exceeds the capabilities of all supercomputers available today, combined. This means that we are currently unable to find explanations for phenomena from the quantum world, including conductivity, magnetism and superconductivity," says Benedikt Fauseweh from the DLR Institute for Software Technology. "Quantum physics is fundamental to understanding our world, especially solid-state physics." In solids, atoms or molecules are so close together that they hardly move at all. Metals, semiconductors, ceramics and many plastics are solids.
A visionary idea – but quantum computers still make mistakes
The concept of simulating quantum phenomena on a quantum computer is more than 40 years old. In 1982, the US-American physicist Richard Feynman (1918 to 1988) proposed using the principles of quantum mechanics itself to simulate the behaviour of quantum systems. “The development of modern quantum computers is bringing Feynman’s visionary idea closer to reality. Of course, it remains the dream of all physicists to describe all of nature in its full complexity,” says Fauseweh. But we are not there yet; quantum computers still make errors in complex calculations because their qubits (computing and storage units) are not stable or cannot be controlled, leading to incorrect results. Nevertheless, researchers are looking for ways to run sophisticated simulations on quantum computers.
Large number of qubits not required
Fauseweh has described this in the scientific journal Nature Communications and included worldwide research results. His analysis shows that a large number of qubits is not necessarily required for the simulation of complex quantum systems: "If we use too many qubits, the results are no longer useful due to errors in the quantum computer," explains Fauseweh. "We have to identify precisely those applications that still deliver a correct result even with errors in the quantum computer." So, with quality before quantity, digital quantum simulations could soon solve the mysteries of solid-state research: why do interactions between many particles prevent their movement in solids? Why, for example, do some materials not heat up as expected in a magnetic field? How do materials change their properties when they are irradiated with lasers? "It is also exciting that we can use quantum computers in materials research to make quantum computers themselves better."
Different technologies for quantum computers certainly complement one another. Photonic quantum computers or quantum computers with neutral atoms, for example, would be particularly suitable for some applications, while quantum computers with ion traps, with nitrogen vacancies in diamond or other solid-state spins, would suit others. These hardware technologies are being developed in the DLR Quantum Computing Initiative (DLR QCI).
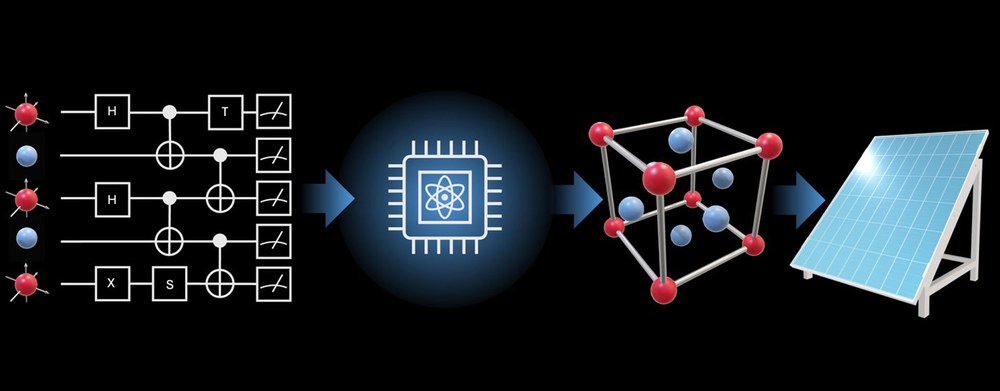
Analogue simulations reach their limit
In recent decades, researchers have used analogue quantum simulations to assist them – with a quantum platform that serves as a substitute for the actual system. The behaviour of the substitute quantum platform is adjusted so that it corresponds to that of the system under investigation. In this way, it is possible to simulate quantum systems that supercomputers had already failed to – and with this the advantages of quantum simulation become apparent. Analogue quantum simulation has been a common method until now. "However, it has the disadvantage that these machines are not flexible. They can only do one type of simulation," says Fauseweh. "What we want is the universality of a digital quantum computer. That is the most important advantage we expect from the different approaches."
Related links
Quantum computers at DLR
Quantum computers are an important technology for the future. They can perform calculations and simulations in specific areas much faster than conventional supercomputers. They can be used in the transport and energy sectors, for example, but also in fundamental research or satellite operations. Quantum computers utilise quantum mechanical effects such as entanglement and superposition. Their quantum bits (qubits) can assume the states 0 and 1 simultaneously – and not just one after the other, like classical computers. This, in turn, is what makes quantum computers so powerful. Several DLR institutes are working with quantum technologies, and there is a great need at DLR to continue to conduct research on and with quantum computers. Quantum computing has incredible potential and is one of the most fundamental and pioneering areas of expertise needed to ensure a leading international position for German industry in the future.