Vehicles and Infrastructure
High-Speed Trains Passing through Tunnels
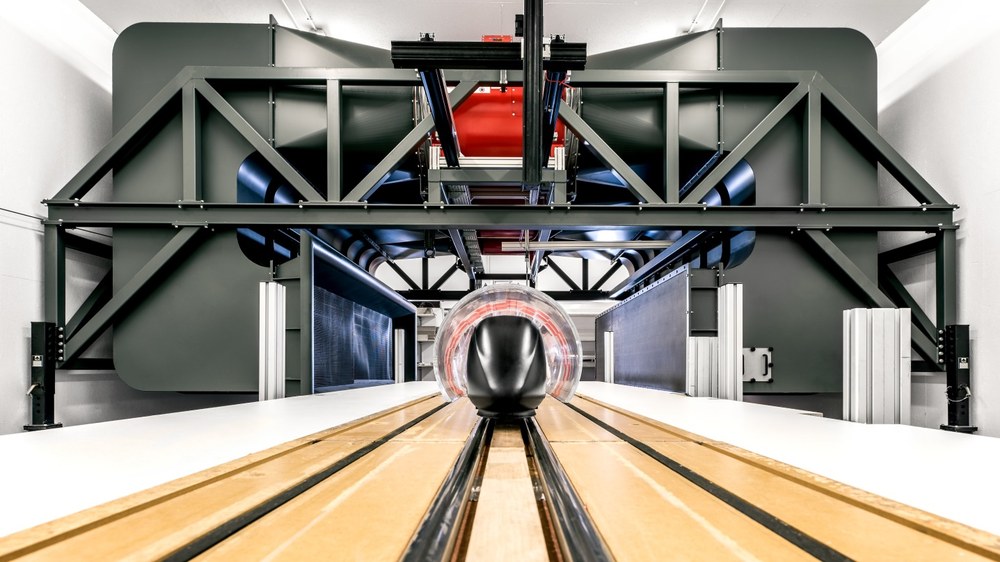
When high-speed trains pass through tunnels, strong pressure waves can be generated causing a variety of negative effects. The structures both of the tunnel and of the train are affected by the strong, sudden pressure changes. The emergency exit doors, for example, must withstand the strong pressure fluctuations and must therefore be regularly serviced, repaired and – if damaged – replaced. Passenger comfort is also influenced by these pressure fluctuations. In addition, the initial pressure wave generated when the train head enters the tunnel can lead to the so-called tunnel boom, a loud banging noise that might disturb potential residents in the area of the tunnel exit.
As a result of modern tunnel construction, where the tunnel consists of two small tubes with one track for each direction instead of one large tube with two tracks, and due to the use of double-decker trains to increase passenger capacity, the problem of pressure waves in railway tunnels will become more relevant in the near future. The increasing blockage of the tunnel cross-sectional area by the large trains and the desired higher train speeds lead to an increase in the pressure amplitude and thus in the loads on the installations in the tunnel.

A slight reduction of the pressure-related, negative effects could be achieved by extending and optimising the geometry of the train head and by reducing the speed at which the train enters the tunnel. However, these measures conflict with the aim of increasing the efficiency of rail transport.
Instead, a tunnel can be equipped with an extended tunnel portal smoothening the pressure increase in the tunnel and thus reducing the risk of damage to installations in the tunnel and the train itself.
With the help of the Tunnel Simulation Facility Göttingen (TSG) countermeasures such as tunnel portals or measures within the tunnel are designed, investigated and evaluated using a variety of measurement techniques including, for example, pressure, force or velocity sensors and optical methods such as Particle Image Velocimetry (PIV). In addition, the pressure waves are simulated and analysed using theoretical models or Computational Fluid Dynamics (CFD). Thus, numerous aerodynamic effects occurring when high-speed trains pass through tunnels can be studied.
Transient Loads on Vehicles

Safety and environmental compatibility are decisive factors when choosing a means of transport. For this reason, mainly trains but also trucks and cars are subject to a large number of tests during the development phase, intended to ensure operational safety in case of strong crosswinds and low energy consumption. Standardised procedures are used to achieve results that are as reproducible as possible.
However, in real operation - unlike investigated during the development phase – transient loads or uneven and sudden gusts can occur, which can rapidly change the crosswind sensitivity of the vehicle, for example. Moreover, the energy requirement of a vehicle can also increase due to the uneven flow.
In order to ensure safe and energy-efficient operation of all types of vehicles, investigating the influence of transient loads is of great importance.

The vehicle can be examined by means of ground simulation using a moving belt system or on a conventional fixed ground. For this purpose, advanced measurement techniques such as scales installed in the model, pressure sensors or optical measurement techniques such as Laser Doppler Anemometry (LDA) or Particle Image Velocimetry (PIV) are available. Road tests with vehicles equipped with state-of-the-art measurement technique are also performed to analyse transient flows or their effect on a moving car, truck or train. In addition to the experiments, the flow around the vehicles is simulated numerically, and the calculated flow fields are then analysed with regard to the respective problem.
Adaptive Train Geometries
Modern trains are aerodynamically optimised in many ways. One focus in the development process is to reduce the energy consumption of the train, which is why bogies, car transitions and the like are often aerodynamically faired on high-speed trains. The shape of the train head is also influenced by this and must be designed in such a way that crosswind loads and consequently the probability of derailment are reduced. However, a high-speed train usually does not have a designated main direction of movement, as it does not turn around in terminal stations or at the final station, for example, but continues in the opposite direction. This means that every train head is also a train end, which makes it difficult to optimise the geometry as the requirements for the head and the end of a train are very different. This is also an issue when two high-speed trains are mechanically coupled to operate in double traction or in the case of virtual coupling, where two trains follow each other directly in an electronically controlled distance far below the breaking distance.

If a train passes through a crosswind field, it experiences an asymmetrical flow. The train head, however, is generally designed symmetrically as the crosswind could come from either side. A train could therefore be operated more safely and efficiently if these aspects were taken into account and the train geometry could be adapted to the external circumstances. In order to identify positions for potential geometric adaptations, the flow pattern can be characterised by means of numerical or experimental procedures. The influence of small changes in geometry can be quantified by numerical methods. Possible changes in geometry include variable spoilers or retractable flaps, but also an adaptive car body that could possibly be expanded or deformed by using new innovative materials.