Concept
Noise abatement is a concern for ATM today – with different level of emphasis depending on the local situation – but only generic procedures are employed that aim at increasing the height of the flight path (raising intermediate altitudes, increasing glide path angle and initial climb angles) and its horizontal distance from noise-sensitive areas. At busy times, there is normally not enough leeway to allow Continuous Descent Operations (CDO) that minimise fuel consumption and engine noise by flying from Top of Descent (ToD) through intermediate approach at near idle power.
Taking into account that during approach airframe noise often dominates engine noise [BAUER and KÖNIG 2016], the overall environmental optimisation is even more complicated. The drag and noise characteristics of the different low-speed configurations of the aircraft, cf. fig 1.2 (below), are such that flying higher or slower than a reference can, contrary to intuition, in fact increase noise immission on the ground (the noise that arrives at a certain point in space, as opposed to the emission, which is the noise generated at the source) as well as fuel consumption and generation of pollutants. Therefore, even without taking (read ATC) restrictions into account and assuming perfect knowledge of the wind situation, it is a challenge in itself to determine and fly a procedure that is simultaneously safe, cost-effective and environmentally friendly [KÖNIG 2010; KÖNIG and KRETH 2014].
In daily practice, such an optimised procedure would require more sharing of information and intent between ATM and the aircraft. From the airborne perspective, the most restricting constraints for an optimised procedure are the lack of information about the exact distance to fly, wind profile characteristics particular with the influence of orographic features, inversion layers etc. and the missing information about the detailed ATM speed schedule, which by itself often depends on the preceding aircraft and the particularities of a specific airport. The DYNCAT project takes up this challenge by studying and quantifying the shortcomings of the current practice and demonstrating through analysis and simulation the potential benefits of improved operations which assume the availability of the above information through novel technologies (including the algorithms developed within the project itself). The Airbus A320 family is chosen as reference aircraft and the optimisation of the high-lift system sequencing during approach constitutes the use case for the project.
Optimisation of high-lift system sequencing and timing is mandatory to make descents and approaches more environmentally friendly, more fuel efficient and minimising the time that aircraft have to be at low altitude, thereby reducing their environmental impact. This kind of operations aims to fly the descent and approach at near idle thrust and the airspeed resulting from the envisaged sink rate. However, controllers often need to use intermediate level-off and speed instructions to ensure the separation. Therefore, the aircraft is no more able to fly its reference profile as it was initially planned. It might be combined with unpredicted wind that decreases the flight predictability. This leads to either low or high energy situations that impact the environment through the use of thrust or speed brakes depending on the case. An efficient way to address the environment uncertainty and the controller’s impacts on the flights is to optimise dynamically the high-lift system sequence.
This sequencing of high-lift system and landing gears is currently based on pilot’s skills only so that it is no wonder that measurements show many flights are over-consuming compared to the optimum [SCHOLZ and SENSKE 2018]. Indeed, pilots need to take some margins in order to avoid over-energy approaches and goaround situations, and this often includes early extension of the landing gear and use of speed brakes, whichincreases the noise footprint unnecessarily.
The latest generation of Flight Management Systems (FMS) still computes a static high-lift sequence with a fixed order that is simplified, considering the landing gears grouped with the two last configurations. Thus, it only permits the display of the flaps 1 and 2 pseudo-waypoints in the cockpits with an associated energy status. Their location corresponds to the theoretical extension linked to the reference profile, and the energy status warns the pilots if the configuration will not be extendable at the pseudo-waypoint sequencing. Thus, it helps the crew in the very specific situation when the aircraft is on path in nominal situation. But with the worldwide traffic increase, there will be more and more aircraft in the vicinity of the airports, the need for ATC instructions to ensure separation will increase and the current capability will not be sufficient.
DYNCAT aims at dynamically computing the high-lift sequence on-board in order to provide the crew with the best strategy for landing. It will incorporate both the available weather information and the controllers’ instructions into the Flight Management System (FMS) to provide decision support-tools to the crew, including the HMI part. It will also determine the best configuration for landing, and the best moment to extend the landing gear. DYNCAT will make extensive use of stakeholder knowledge, especially from the operational side, in involving active air traffic controllers and airline pilots in the design and evaluation of the proposed functionality.
The demonstration activity for an improved functionality is preceded by an in-depth analysis of the current situation and identification of the potential for improvement. The influence of ATC instructions on the operational feasibility and environmental friendliness of a landing approach is not only highlighted but also quantified through analysis of measurement data for current operations and high-fidelity simulation. Hands-on workshops on intermediate and final concepts and their implementation with operational air traffic controllers and pilots subcontracted for this purpose, to which participation of representatives from the further stakeholder groups will be invited, will ensure making utmost use of stakeholder knowledge.
These activities allow delivering a concept outline and identification of potential benefits and risks and advancing the Technology Readiness Level (TRL) from pre-TRL 1 to 3. DYNCAT has an ambitious target TRL for Exploratory Research, reachable because the proposed functionality is an extension of capabilities of the current FMS and thus its early prototype implementation can be integrated into an existing hardware test environment. The characteristic proof-of-concept verification of technical feasibility of DYNCAT’s early prototype implementation will be exercised in human-in-the-loop real-time simulations with representative data. DYNCAT’s results will be able to be picked up directly in Industrial Research follow-up projects.
DYNCAT will benefit from the results of prior research including the observations made from flight tests under operational conditions. Specifically, there will be a close relation with various ongoing national and international projects evaluating the Low Noise Augmentation System (LNAS), (FluiD21 – flight mechanical optimisation of Continuous Descent Approaches) and (SIAM – quiet mid-range airliner) DLR internal projects. Concerning SESAR, the activities mentioned in section 1.2 will be monitored although there is no direct input to DYNCAT. From THALES AVS side, DYNCAT we be included in the FMS product policy and considerably complements and improves the Continuous Descent Approach project.
All the activities will help to better understand and demonstrate how the environmental impacts of ATM operations can be reduced during the approach flight phase, in TMAs.
References:
[BAUER and KÖNIG 2016] Bauer, T. and König, R., Lärmreduzierte Anflugprofile – Verfahren und Umsetzungspotentiale (Noise Reduction Approach Profiles – Procedures and their Implementation Potentials), DLR-IB 111-2015/30, Braunschweig: 2016.
[KÖNIG 2010] König, R.: Operational Evaluation of Steeper Final Landing Approaches, International Conference on Active Noise Abatement, 23.-24. September 2010, Frankfurt/Main, 2010.
[KÖNIG and KRETH 2014] König, R.; Kreth, S.: Untersuchungen zum Einfluss des Fluggeschwindigkeitsprofils auf Fluglärm, Treibstoffverbrauch und Flugzeit im Endanflug (Study of the Influence of the Airspeed Profile on Aircraft Noise, Fuel Consumption and Flight Duration on Final Approach). DLR-IB 111-2014/49. Braunschweig: 2014.
[SCHOLZ and SENSKE 2018] Scholz, M. and Senske, V., Auswirkungen unterschiedlicher Anflugverfahren auf den Treibstoffverbrauch auf Grundlage operationeller Flugbetriebsdaten (Effects of Different Approach Procedures on Fuel Consumption based on Flight Operational Data). German Aerospace Congress (DLRK), Friedrichshafen: 2018.
This project has received funding from the SESAR Joint Undertaking under the European Union's Horizon 2020 research and innovation programme under grant agreement No 893568.
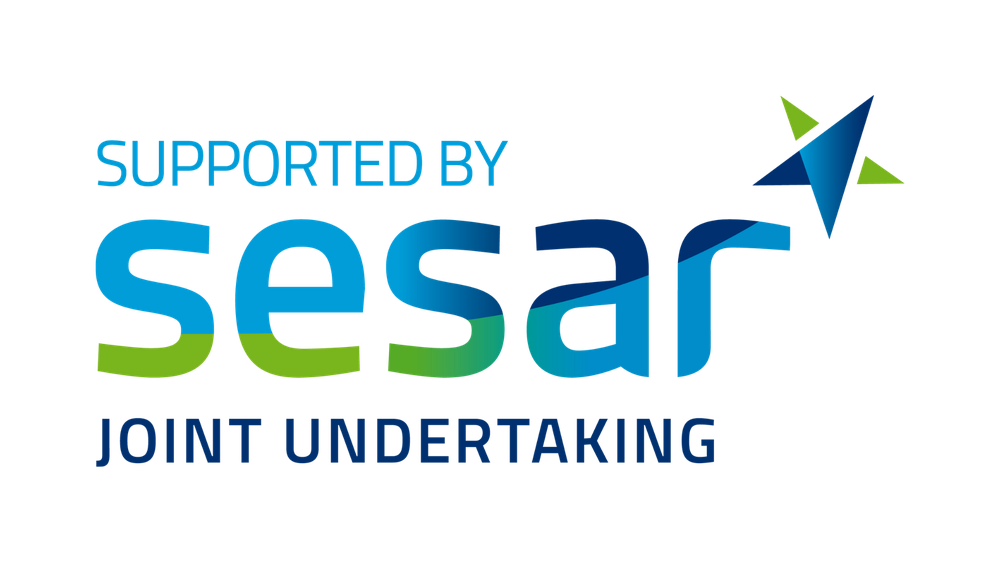
SESAR 3 Joint Undertaking

Copyright DYNCAT Consortium 2020-2022. All rights reserved. Licensed to the SJU under conditions.