MMX rover simulation – realistically recreating the conditions on Phobos
Driving in a low-gravity environment poses a special challenge for the MMX rover because the gravitational pull of Phobos is about two thousand times less than that of Earth. Therefore, many tests cannot be carried out on Earth in a representative environment. Instead, DLR has developed detailed simulation models of the rover. This allowed the researchers to check critical phases and test software in a virtual environment.
Why is simulation so important for the MMX rover?
NASA's 'Curiosity' and 'Perseverance' rovers are on exploration missions on Mars. Since the gravitational pull on Mars is about one-third that of Earth, it is possible to conduct representative tests on Earth with a functionally identical rover that weighs only one-third of 'Curiosity' or 'Perseverance'. In the California desert, NASA researchers can test whether the rover can cope with a slope and get safely through difficult terrain or get over an area of particularly soft sand. Such tests are not possible in the MMX project. Since the gravitational pull on Phobos is two thousand times less than on Earth, a rover two thousand times lighter than the MMX rover would have to be used for a representative test.
Testing in a drop tower or parabolic flight was also not an option in the development of the MMX rover. The effective time without gravity – four seconds in the drop tower or about 20 seconds in parabolic flight – is too short to perform a measurement of a wheel spinning through a sand field, for example. Moreover, for a representative test, the sandy soil would have to be loosened before a wheel test could be carried out.
Although all functions, such as deployment of the solar panels and the running gear settings, can be examined in laboratory experiments, it cannot be guaranteed that they will work in the same way on Phobos. However, this is of great importance, especially in critical phases such as during landing, uprighting and alignment with the Sun.
This is where simulation comes into play. Gravitational acceleration is just one parameter among many that can be adjusted. This allows researchers to extrapolate from the laboratory tests to the conditions on Phobos – in other words, they can make predictions about situations that lie outside what is known so far. Another big advantage of the simulation is that all variables are visible, even those that cannot be measured with sensors on the hardware, such as the exact speed of the rover or the sinking of the wheels into the ground. In addition, a simulation can be repeated as often as desired, for example to examine the influence of a parameter on the entire system in isolation. In addition, a large number of similar tests can be carried out in parallel.
Details of the simulation model
The simulation model as a whole contains the mechanical model of the rover, complete with wheels, actuators, mechanisms and sensors, the model of the environment (ground geometry and properties) and the contact mechanics as a connection between the two, as well as a control unit for the actuators. In addition, there are a number of other elements for setting up and evaluating test series and a 3D visualisation.
The centrepiece of the simulator is a detailed mechanical model. The exact masses and dimensions of the construction are depicted, as are the shafts and gears used to transmit power between the motors and wheels. Mechanisms for deploying the solar panels, for example, are also modelled faithfully. Sensors, which also exist in hardware, are also present, with optional effects such as noise and drift. The researchers use Modelica as the modelling language and Dymola as the environment for developing and running the simulations.
Orbiter missions to Mars, such as the European Mars Express orbiter, allow conclusions to be drawn about the ground conditions on Phobos – for example, how steep the slopes are, how coarse the sand is and what the average size of the stones is – but the resolution of the images is not high enough. The simulation model must therefore be able to create soil geometry and rocks according to the best statistical parameters determined by planetary scientists. A random generator then creates a set of different environments on which the same test takes place. This can be used to estimate how well, on average, the rover will achieve its goals.
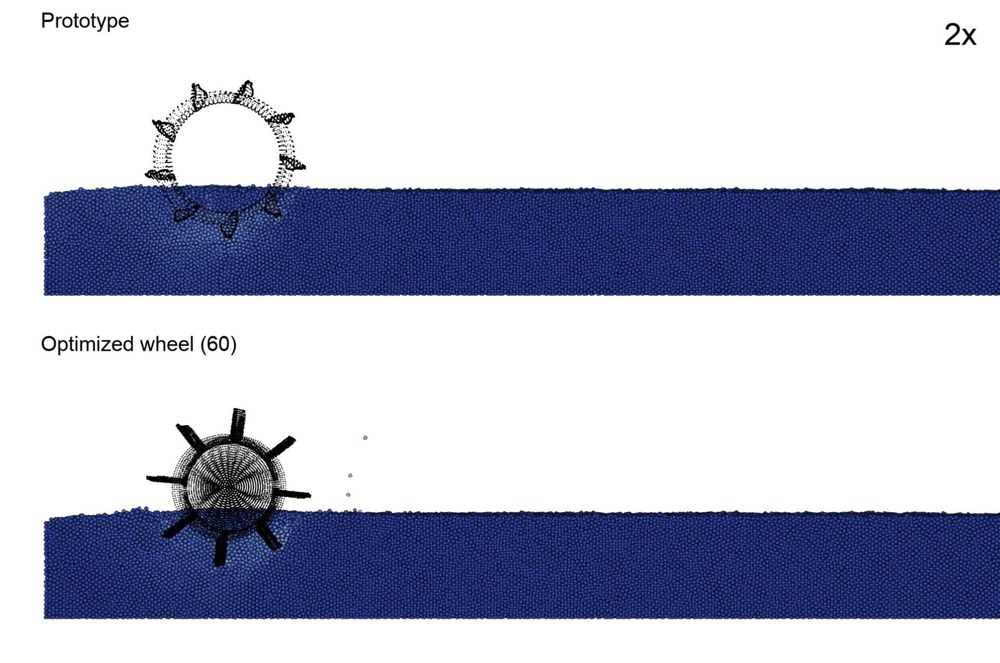
Animation: DEM simulations for optimising the wheels of the MMX rover
Your consent to the storage of data ('cookies') is required for the playback of this video on Quickchannel.com. You can view and change your current data storage settings at any time under privacy.
Applications
Uprighting
Before the rover can travel on Phobos, it must survive the landing and orient itself, because the legs and solar panels are folded during the journey from Earth to Phobos.
While the rover will survive the landing relatively safely due to the design of the chassis and wheels, the uprighting phase is particularly critical. The rover has to turn onto its 'belly', 'stand up' on its legs and deploy the solar panels. All this has to happen fully autonomously, because for one thing, the signal travel time between Phobos and Earth is several minutes, and there is no constant visual and radio contact. For another, the antenna is located under the folded solar panels, so good contact can only be established after the deployment phase. The simulation was also a crucial tool to develop the best sequence of commands for the legs so that the uprighting succeeds in most cases.
The researchers also had the idea of equipping the rover with a mechanism to help it tip over in the event of an unfavourable starting position. However, series of simulations have shown that this approach would do more harm than good – the mechanism could block the solar panels in case of a defect. To come to this conclusion, the researchers ran many similar simulations in parallel, each with a slightly different environment or different initial conditions, so that finally a statistically sound statement could be made about the success or failure of the uprighting process. Following the simulation results, the additional mechanism was ultimately not integrated into the rover.
Driving
The most important task of the MMX rover will be to drive on Phobos. On the martian moon, the rover will make the very first attempt at wheel-driven locomotion in milligravity. Simulation is the only way to get a good estimate of how fast the rover can safely travel without losing contact with the ground.
Equally important are studies on maximum acceleration and slip, for example during turning manoeuvres. This is because the rover has no actuators to turn the wheels to the right or left. The rover makes turns by turning the wheels on one side faster than on the other – in extreme cases also in opposite directions, in which case the rover is supposed to turn on the spot. For the sandy soil that is to be expected on Phobos, the simulation showed that a command to the wheels that would correspond to a turn of 90 degrees with zero slip – that is without spinning the wheels – only produces a turn of 35 to 55 degrees.
These and other results of the simulation are already important for hardware and software development and will also be useful later in the operations phase. With the telemetry data from the operations phase, the researchers will also go the other way and validate the simulation models for the actual conditions on Phobos. The on-site measurements and the validated simulation models will provide valuable scientific knowledge about Phobos.
MMX – Martian Moons eXploration
MMX is a mission of the Japanese space agency JAXA with contributions from NASA, ESA, CNES (the French space agency) and DLR. CNES (Centre National d'Études Spatiales) and the German Aerospace Center (Deutsches Zentrum für Luft- und Raumfahrt; DLR) are jointly contributing a 25-kilogram rover to the Martian Moons eXploration Mission (MMX). The Franco-German MMX rover is being designed and built under the joint leadership of CNES and DLR. In particular, DLR is responsible for the development of the rover's landing gear, including the lightweight body, as well as the entire uprighting and locomotion system. DLR is also contributing the connection adapter to the MMX spacecraft and providing a Raman spectrometer and a radiometer as scientific experiments. These will analyse the surface composition and texture on Phobos. CNES is making significant contributions with camera systems for spatial orientation and exploration on the surface, as well as for the study of mechanical soil properties. CNES is also developing the rover's central service module, including the on-board computer and the power and communications system. After the launch of the MMX mission, the rover will be operated by CNES control centres in Toulouse (France) and DLR in Cologne (Germany).
For DLR, the institutes of System Dynamics and Control, Composite Structures and Adaptive Systems, of Space Systems, of Optical Sensor Systems, of Planetary Research, for Software Technology and the Microgravity User Support Center (MUSC) are also involved under the leadership of the DLR Institute of Robotics and Mechatronics.
The MMX mission is a continuation of an already long-standing successful cooperation between JAXA, CNES and DLR. It builds on the previous mission Hayabusa2, in which JAXA sent a spacecraft to the asteroid Ryugu with the German-French MASCOT lander on board. On 3 October 2018, MASCOT landed on Ryugu and sent spectacular images of a landscape ridden with boulders and rocks, and virtually no dust. Hayabusa2 collected samples from Ryugu and brought them to Earth on 6 December 2020.